Editor's note:Yellowstone Caldera Chronicles is a weekly column written by scientists and collaborators of the Yellowstone Volcano Observatory. This week's contribution is from Dr. Madison Myers, Associate Professor of Earth Sciences at Montana State University, Stacy Henderson, PhD student at Montana State University, and Dr. Colin Wilson, Professor at the Victoria University of Wellington, NZ.
If you are a fan of Yellowstone’s volcanic history, you have probably seen the simplistic ashfall map that highlights how, during its largest eruptions, ash is distributed (and mostly preserved) across the United States. However, you might not know how these maps are created, or how volcanologists use these data to determine the height of the eruption plume produced during a caldera-forming eruption. To explore this question, we’ll discuss how volcanologists study ash deposits and take a short field trip to a sedimentary basin in central Wyoming, where geologists have found something remarkable.

Map of volcanic ashfall. Areas of the United States that once were covered by volcanic ash from Yellowstone's giant eruptions 2 million and 630,000 years ago, compared with ashfall from the 760,000-year-old Long Valley caldera eruptions at Mammoth Lakes, California, and the 1980 eruption of Mount St. Helens, Washington/Used with permission from "Windows into the Earth, The Geologic Story of Yellowstone and Grand Teton National Park", Robert B. Smith and Lee J. Siegel, Oxford University Press, 2000.
First off, what is an ash plume? In volcanic systems, these are mixtures of gas, ash, rocks, and crystals that are released from an eruptive vent at speeds that approach or exceed the speed of sound (343 meters per second, or 767 miles per hour). For smaller plumes, the wind controls the ash plume’s pathway, resulting in ash that falls in an elongated zone downwind of the volcano, like that of the 1980 Mount St. Helens eruption. However, as the eruption size grows, often so does the plume height, leading to plumes that are able to spread-out like an umbrella and deposit ash over broader areas. A recent example of a powerful umbrella-like plume was the 2022 eruption of Hunga Tonga in the south Pacific—an event that could be seen clearly from space.

Simplified schematic of a volcanic plume ejecting ash, crystals and fragments of rock from a vent. This rising plume will eventually hit a zone of neutral buoyancy in the atmosphere, where it is then carried by the wind. Material is ejected from both the upward moving jet and falls from the umbrellaing plume/Modified from Wilson and Houghton (2000), Encyclopedia of Volcanology first edition.
Although caldera-forming explosive eruptions are amongst the most devastating natural events on Earth, they are not common. Thus, the tools available to determine the height of the ash plumes of past eruptions rely on measuring the thicknesses of their ash-fall deposits and sizes of their particles. Essentially, if a plume is larger, it will leave thicker deposits at greater distances from the source vent, with pumice (shattered magma) and lithics (rocks torn from the vent) that become gradually smaller with distance. By measuring these parameters in the field at numerous locations around the vent, scientists can estimate the height of the eruption plume.
If there are discrete layers in the ash deposits, this technique can even be used to tell if the plume height changed through time! For instance, scientists can see that the major eruption that occurred about 3,600 years ago from Santorini Volcano, Greece, started with a 10 km (6 mi) high plume that grew to 30 km (19 mi)—for reference, the cruising altitude of most airplanes is 9–12 km (30,000–40,000 feet). This is why calculating plume heights from past eruptions is important for understanding the potential impacts of future eruptions on aviation. For a volcanic eruption the size of Yellowstone’s largest caldera-forming events, the plume likely reached the top of the stratosphere, which is 50 km (31 mi) above the Earth’s surface!
In the 1960s, geologists Ray Wilcox and Glen Izett created ashfall maps for past Yellowstone eruptions by driving around the western United States, identifying Yellowstone eruption deposits based on their chemistry, and measuring characteristics like thicknesses. These maps were instrumental in understanding the characteristics of eruption plumes from Yellowstone’s major explosive eruptions. If you live in any of the many states covered by these deposits, you can visit these locations by looking them up on the map that Wilcox and Izett created, which includes coordinates and field descriptions (https://pubs.usgs.gov/publication/i1325).
A group of geologists from Montana State University and Victoria University of Wellington (New Zealand) recently visited one such location near Shell, Wyoming. At this site, two ash fall deposits associated with the two defined ash flow units of the Lava Creek Tuff, which resulted from the formation of Yellowstone Caldera about 631,000 years ago, have been reported. But the geologists found something else as well. Not only did this basin, tucked against the Bighorn Mountains, contain the deposits in question, but beneath the Lava Creek Tuff deposit, and therefore older in age, were two additional ash fall deposits that are not in Wilcox and Izett’s maps!
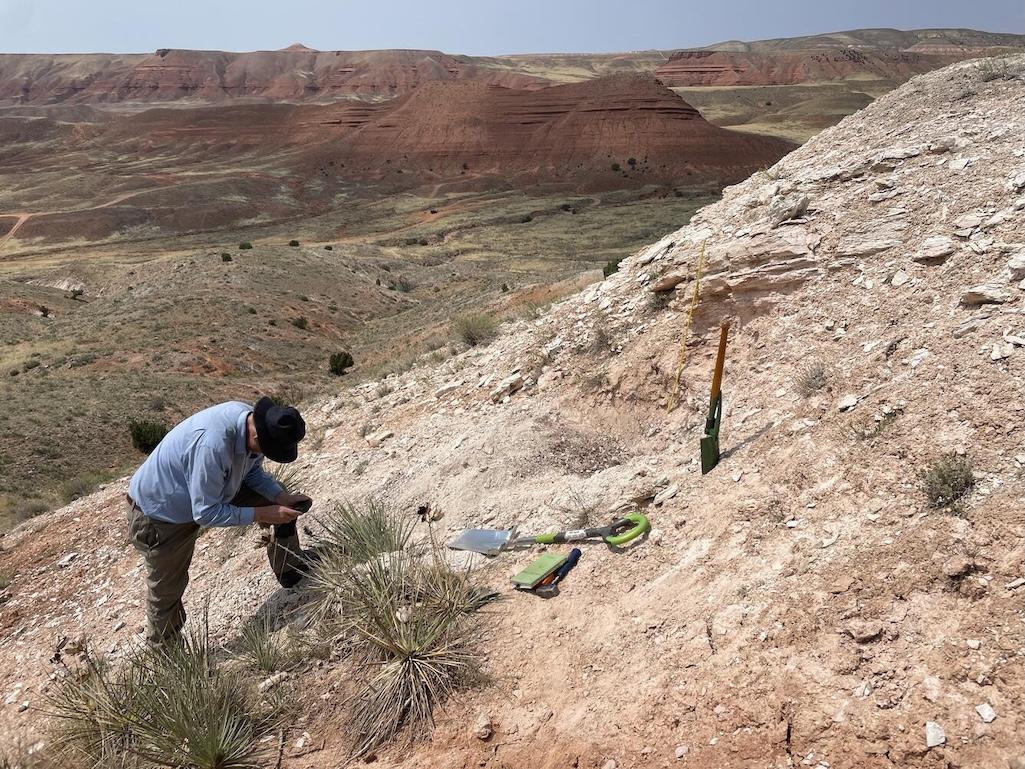
Professor C.J.N. Wilson, FRS, pays due homage to the Lava Creek Tuff ashfall bed in a basin just east of Shell, Wyoming/Madison Myers, Montana State University, August 9, 2024.
What are some possible sources of thick additional ash in the middle of Wyoming? Could they be the ashfall deposits from the Mesa Falls Tuff (1.3 million years old) or Huckleberry Ridge Tuff (2.1 million years old), also from Yellowstone? Or could it even be ash from farther away, for instance, the Bishop Tuff eruption, which formed Long Valley Caldera, California, about 767,000 years ago? The presence of crystals of the mineral biotite in the one of the mystery deposits points toward the Bishop ash as a likely suspect, as this mineral is not associated with any of Yellowstone’s major eruptions. But what about the older ash? To settle the debate, geologists sent samples of the mineral sanidine from each of these deposits to the US Geological Survey at Moffett Field, California, for dating using the argon geochronology technique. The results will give the ages of the eruptions that fueled these ash deposits, thus telling geologists the likely sources. We don’t yet have the answer, but will report back once the results are in.
Although the mystery regarding the source of the unknown ash beds will soon be solved, another mystery will remain: how did this basin in central Wyoming preserve so much volcanic ash, both from Yellowstone and perhaps beyond?